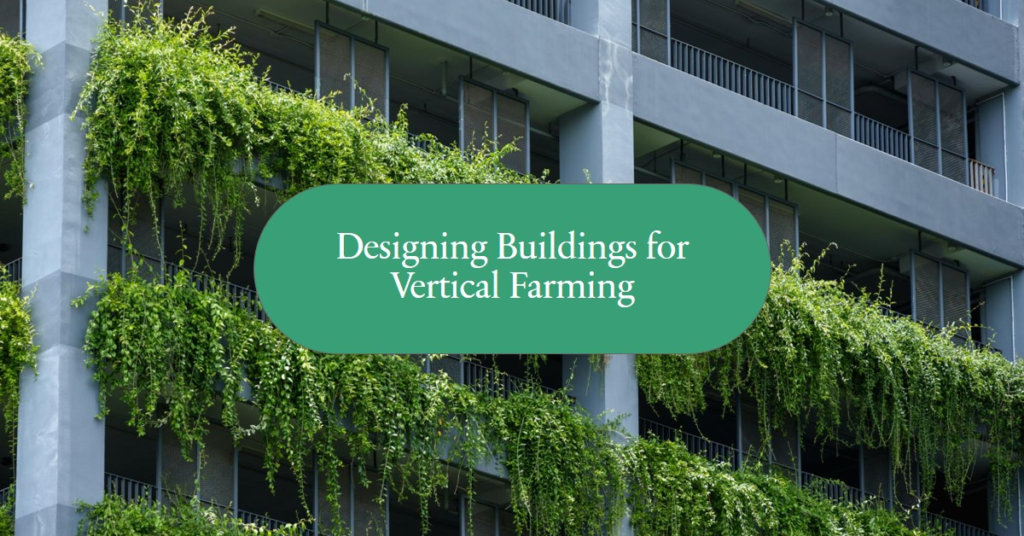
Table of Contents
- Introduction to Vertical Farming
- Architectural Constraints and Considerations
- Energy Efficiency and Lighting
- Water Management Systems
- Climate Control and Ventilation
- Space Optimization and Plant Density
- Integration of Technology and Automation
- Case Studies and Real-World Examples
Introduction to Vertical Farming
Vertical farming stands as a revolutionary approach in modern agriculture, addressing the multifaceted challenges of traditional farming. At its core, vertical farming involves the cultivation of crops in stacked layers, often integrated into other structures like skyscrapers, repurposed warehouses, or shipping containers. This innovative technique enables the growth of large quantities of produce within a reduced spatial footprint.
The significance of vertical farming is underscored by its potential contributions to sustainable agriculture. As urbanization accelerates and arable land becomes increasingly scarce, vertical farming emerges as a viable solution for enhancing food security. By leveraging controlled environments, vertical farms can maintain optimal growing conditions year-round, independent of external climatic variations. This ensures a consistent supply of fresh produce, reducing reliance on seasonal crops.
Moreover, vertical farming contributes significantly to the reduction of land use. Traditional agricultural practices require vast expanses of fertile land, often leading to deforestation and habitat destruction. In contrast, vertical farming’s vertically-stacked systems utilize significantly less land area, preserving natural ecosystems and promoting biodiversity. This innovative farming model also aids in mitigating urban sprawl, as it integrates seamlessly into existing urban infrastructures.
Transportation costs and associated environmental impacts present another critical concern that vertical farming effectively addresses. Conventional agriculture often incurs substantial expenses and carbon emissions from transporting produce over long distances. Vertical farming, typically situated closer to urban centers, substantially reduces the need for extensive transportation networks. By producing food nearer to the point of consumption, this model minimizes transit times, curtails greenhouse gas emissions, and ensures fresher produce for consumers.
Overall, vertical farming represents a pioneering step towards a more sustainable and resilient agricultural system. Its ability to optimize space usage, bolster food security, and decrease environmental footprints positions it as a crucial component in the future of global food production.
Architectural Constraints and Considerations
Designing buildings specifically for vertical farming presents a unique set of architectural constraints and considerations. One of the primary challenges is the need for high ceilings. Vertical farming typically involves stacking multiple layers of plant beds, which necessitates sufficient vertical space. High ceilings are essential to accommodate these layers, along with vertical farming infrastructure such as lighting, irrigation systems, and environmental control equipment.
Another critical consideration is the strength and flexibility of flooring. Buildings housing vertical farms must support substantial loads due to the weight of plant beds, water, and growing mediums. The floors must be robust enough to withstand this weight, while also being adaptable to the potential reconfiguration of the farm layout. Reinforced flooring materials and construction methods are often required to meet these structural demands.
Adequate space allocation is another key factor. Architectural designs must ensure there is sufficient room not only for the plants but also for the technological infrastructure that supports vertical farming. This includes space for automated systems, lighting setups, climate control, and access pathways for maintenance and harvesting. Efficient use of space enhances productivity and operational efficiency of vertical farming setups.
Building code requirements can also present significant challenges. Many existing regulations were not developed with vertical farming in mind, leading to potential conflicts. For example, requirements for floor load capacities, fire safety measures, and accessibility can impact the design and layout of vertical farms. Navigating these regulations demands careful planning and sometimes the need for seeking variances or amendments to standard building codes.
Addressing these architectural constraints and considerations is essential for the successful implementation of vertical farming projects. It requires collaboration between architects, engineers, and vertical farming experts to create designs that meet structural, spatial, and regulatory needs, ensuring that the innovative potential of vertical farming can be fully realized.
Energy Efficiency and Lighting
The design of buildings for vertical farming presents a unique set of challenges, particularly concerning energy consumption. One of the primary factors driving energy costs in vertical farming operations is the lighting system. Traditional agriculture relies on natural sunlight, but vertical farms often depend heavily on artificial lighting to ensure plant growth. The types of lighting systems used can vary, including fluorescent lighting, high-pressure sodium (HPS) lamps, and light-emitting diodes (LEDs), each with distinct energy requirements.
Fluorescent lighting, while relatively inexpensive, tends to be less efficient and has a shorter lifespan. HPS lamps offer greater intensity but also consume substantial amounts of electricity and generate a significant amount of heat, potentially leading to additional cooling costs. In recent years, LED lighting has emerged as a preferable alternative for vertical farms. LEDs are more energy-efficient, produce less heat, and have a longer operational life. Additionally, LED systems can be fine-tuned to emit specific wavelengths optimal for plant growth, further enhancing their efficiency.
To mitigate the energy challenges associated with vertical farming, several strategies can be employed. Firstly, the integration of LED lighting systems can substantially reduce energy consumption while maintaining the necessary light levels for crop growth. LEDs not only reduce electricity costs but also diminish the need for extensive cooling systems due to their lower heat emission.
Another innovative solution is the incorporation of renewable energy sources, such as solar panels or wind turbines, to power vertical farming operations. By generating electricity on-site, vertical farms can reduce their dependence on conventional power grids and cut overall energy costs. Smart grid technology can further optimize energy usage by balancing power consumption and reducing wastage.
Moreover, advances in building design can also contribute to energy efficiency. Utilizing reflective materials and strategically placing windows can maximize natural light penetration, thereby decreasing the reliance on artificial lighting during the daytime. Combining these technologies and approaches can significantly enhance the sustainability and cost-effectiveness of vertical farming systems, making them a viable solution for future food production.
Water Management Systems
One of the key challenges in vertical farming is ensuring effective water management to sustain plant growth. Unlike traditional farming methods that rely heavily on rainwater and groundwater, vertical farming requires innovative water management systems due to its enclosed environment and multi-story structure. Key water management systems utilized in vertical farming include hydroponics, aquaponics, and aeroponics, each offering distinct advantages and infrastructure considerations.
Hydroponics is a system where plants are grown in a nutrient-rich water solution, eliminating the need for soil. This method allows for precise control over nutrient levels and water usage, essential in an urban setting where resources may be scarce. Efficient water recycling is facilitated through closed-loop systems that minimize waste. However, implementing hydroponics at a multi-story level demands a robust and sustainable infrastructure, including water pumps, filtration units, and appropriate plumbing to ensure each tier receives adequate water and nutrients.
Aquaponics, a combination of hydroponics and aquaculture, leverages the symbiotic relationship between fish and plants. Fish waste provides natural fertilizers, while plants help to filter and purify the water. This system requires additional infrastructure, such as fish tanks and biofilters, to maintain water quality. Furthermore, balancing the needs of both fish and plants within a multi-story building adds complexity to the design and operational management.
Aeroponics, on the other hand, grows plants in an air or mist environment without the use of soil or an aggregate medium. Roots are periodically misted with a nutrient solution, making water usage extremely efficient. The infrastructure for aeroponics includes misting devices, fine-tuned nutrient delivery systems, and environmental control systems to maintain optimal humidity levels. While aeroponics can significantly reduce water consumption, it also necessitates highly sophisticated technology and real-time monitoring to ensure system reliability, particularly in a multi-story arrangement where access can be constrained.
Designing buildings to effectively support these water management systems in vertical farming is a complex challenge. Engineers and architects must collaborate to integrate state-of-the-art irrigation systems, advanced sensors, and automated control mechanisms within the building’s framework. These systems not only aim to reduce water wastage but also ensure a continuous supply of water and nutrients at all levels of the structure, thereby enhancing the overall efficiency and sustainability of vertical farming practices.

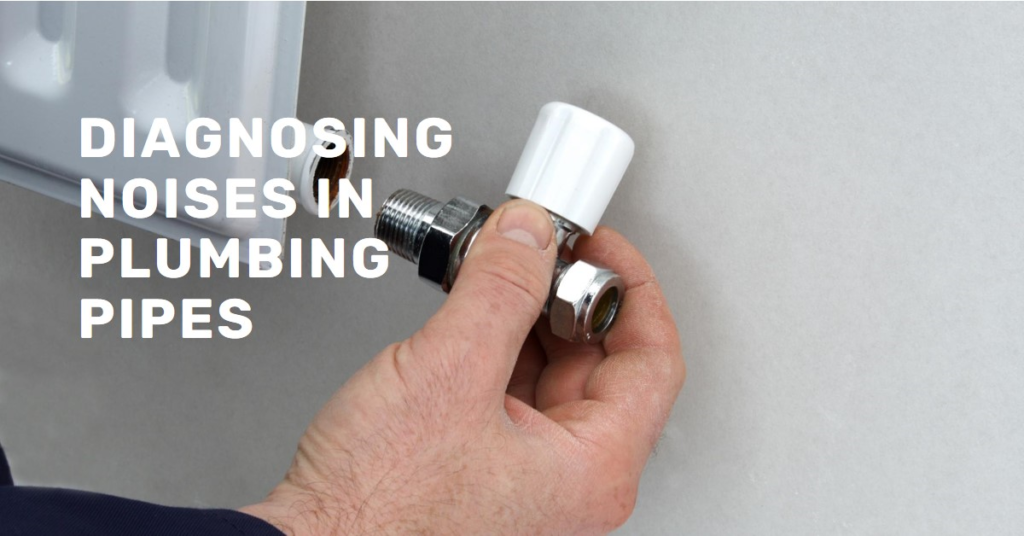
Climate Control and Ventilation
Maintaining optimal climate conditions within a vertical farming setup is fraught with numerous challenges, given the necessity to replicate the environmental conditions typically found in traditional agricultural settings. Achieving the perfect balance of temperature, humidity, and CO2 levels is crucial for the growth and yield of crops. These controlled environments require advanced Heating, Ventilation, and Air Conditioning (HVAC) systems tailored specifically for vertical farming.
One major difficulty in vertical farming is temperature regulation. Diverse crops have particular temperature requirements, necessitating precise and adaptable climate control to prevent fluctuations that could compromise plant health. High temperatures can accelerate plant metabolism, potentially leading to suboptimal growth, while low temperatures can slow it down. Advanced HVAC systems must, therefore, be calibrated to maintain consistent temperatures across vertically stacked layers in these urban farms.
Humidity control is another critical factor. Excessive humidity can promote the growth of mold and mildew, endangering the crops, whereas insufficient humidity can lead to desiccation and stress-induced damage. Hence, the deployment of sophisticated humidity control systems is indispensable. These systems monitor and adjust humidity levels, thereby ensuring an ideal environment for plant growth.
Moreover, CO2 supplementation plays a significant role in enhancing photosynthesis, leading to better plant growth and productivity. In enclosed vertical farming structures, natural CO2 exchange is limited. Thus, CO2 levels must be artificially increased to meet the plants’ needs. Precision climate control systems, integrating CO2 sensors and regulators, can facilitate optimal CO2 enrichment, thereby boosting crop yields.
Addressing these complexities, innovative solutions involve using precision climate control systems and advanced ventilation designs tailored for vertical farming. Such systems enable real-time monitoring and adjustments, ensuring environmental parameters remain within optimal ranges. Enhanced ventilation designs promote effective air circulation, mitigating the risks associated with stagnant air and localized climate disparities within the stacked layers.
Space Optimization and Plant Density
In the burgeoning field of vertical farming, space optimization and plant density are pivotal to maximizing yields and ensuring operational efficiency. The innovative utilization of rack systems and rotating shelves exemplifies modern efforts to make the most of available space within vertical farms. By employing multi-tiered rack systems, vertical farms can enhance their plant-growing capacity without the need for extensive horizontal layouts. This multi-layered approach not only conserves floor area but also creates a dynamic environment that can be adjusted to suit the specific needs of various plant species.
Rotating shelves represent another breakthrough in space management within vertical farming. These systems allow plants to receive uniform light exposure and air distribution, crucial elements for robust plant health. With rotating shelves, every plant has the opportunity to thrive, as they rotate in and out of optimal light and airflow zones. Moreover, integrating automated systems to manage these rotating shelves can greatly reduce labor costs and improve consistency in plant production.
Equally important is the consideration of plant spacing. Proper plant spacing is integral to fostering healthy growth and maximizing productivity. Overcrowding can lead to competition for essential resources such as light, water, and nutrients, which can stunt plant growth and reduce yields. Conversely, adequate spacing enables each plant to receive the necessary resources to flourish. This balance is often achieved through meticulous planning and the use of advanced software to simulate and optimize plant layouts within the farm’s vertical structure.
Innovative design solutions also play a critical role in optimizing space. For instance, utilizing reflective surfaces can help channel light to lower levels of the farm, ensuring that all plants, regardless of their position, receive sufficient illumination. Additionally, implementing modular designs allows for easy reconfiguration and expansion of the farming sections as needed, providing flexibility and scalability to accommodate various crop types and volumes.
Overall, the strategies of employing rack systems, rotating shelves, and ensuring optimal plant spacing are fundamental to the successful operation of vertical farms. By leveraging these innovative methods, vertical farms can achieve significant improvements in space utilization, resource efficiency, and plant productivity, solidifying their position as a viable and sustainable solution for future agriculture.
Integration of Technology and Automation
The successful integration of technology and automation in building designs for vertical farming is pivotal for optimizing efficiency and productivity. Vertical farming hinges on a variety of advanced technologies that streamline operations and ensure optimal growing conditions. One of the core components in this regard is the use of Internet of Things (IoT) devices. These devices enable real-time monitoring and data collection, allowing farmers to track and adjust environmental parameters such as temperature, humidity, light, and nutrient levels seamlessly. This constant feed of data is crucial for creating the perfect environment tailored to the specific needs of various crops.
Automated irrigation systems further enhance the efficiency of vertical farming setups. By precisely controlling the delivery of water and nutrients, these systems ensure that plants receive exactly what they need when they need it, reducing waste and promoting healthier growth. Such systems can be programmed to adjust dispensations based on the data gathered from IoT devices, ensuring a synergistic approach to crop management.
Moreover, the implementation of robot-assisted planting and harvesting addresses the labor-intensive nature of traditional farming. Robotics can be programmed to perform a range of tasks from planting seeds to harvesting mature crops with minimal human intervention. This not only reduces labor costs but also minimizes the risk of human error, ensuring consistent quality and productivity.
To support these advanced technologies, buildings designed for vertical farming require a robust and adaptable infrastructure. Infrastructure must facilitate seamless connectivity for IoT devices, a reliable power supply to support automated systems, and appropriate space to house robotic equipment. Additionally, integrating renewable energy solutions such as solar panels or wind turbines can enhance sustainability and reduce operational costs.
In conclusion, integrating technology and automation into building designs for vertical farming offers a path towards highly efficient and sustainable agricultural production. By leveraging IoT devices, automated irrigation systems, and robotic assistance, vertical farms can achieve optimal growth conditions while minimizing resource expenditure and labor costs. As the vertical farming industry continues to evolve, the integration of these technologies will become increasingly essential.
Case Studies and Real-World Examples
Examining real-world examples of vertical farming projects provides invaluable insights into both the challenges and solutions associated with this innovative form of agriculture. One such noteworthy project is the Sky Greens farm in Singapore. Faced with limited land resources, Sky Greens utilized a vertical farming system that sprawls upward instead of outward, optimizing space while maintaining high productivity. By using a unique rotating system powered by water, they significantly reduced energy consumption, showcasing the potential of sustainable practices within urban environments.
Another prominent example is the AeroFarms project in Newark, New Jersey. AeroFarms transformed a former steel mill into a vertical farm, overcoming challenges related to soil contamination and industrial pollution. To address these issues, they developed an aeroponic system that grows crops in a mist environment, eliminating the need for soil. This method not only bypasses the problems associated with contaminated land but also uses up to 95% less water than traditional farming methods.
In Tokyo, the Pasona Urban Farm, nestled within an office building, highlights a unique integration of agriculture into a corporate setting. The main challenge was balancing the needs of a functional office space with the demands of a vertical farm. By using advanced hydroponic technology and careful environmental controls, Pasona was able to create a productive farming environment that also contributes to the aesthetic and air quality of the workplace.
The Infarm initiative in Berlin offers another compelling case study. Infarm installs modular vertical farms within supermarkets, allowing produce to be grown where it is sold. This model addresses logistical and freshness issues by significantly reducing the distance between farm and consumer. The modular system also provides a flexible solution that can be adapted to varying store sizes and layouts, showcasing the versatility of vertical farming in different contexts.
These case studies underscore not only the diverse applications of vertical farming but also the myriad of solutions developed to overcome specific urban agriculture challenges. Each project provides practical insights and illustrates the potential of vertical farming to revolutionize agricultural practices in urban settings, paving the way for sustainable and efficient food production in the future.